Research - Modern Phytomorphology ( 2021) Volume 15, Issue 3
Enzymes of flavonoid biosynthesis as a reason for the polyvariant nature of their accumulation
Ivanov Vyacheslav1* and Shcherbakov Arkadiy22Department of Biological Sciences, Institute of Genetic and Tissue Engineering, Mendeleeva Str. 9, 450022, Ufa, Russia
Ivanov Vyacheslav, Department of Pedagogical Sciences, Nizhnevartovsk State University, Lenina Str. 56; 628605,Nizhnevartovsk, Russia, Tel: 79129385128, Email: karatazh@mail.ru
Received: 14-Apr-2021 Accepted: 28-Apr-2021 Published: 06-Apr-2021, DOI: 10.5281/zenodo.5801114
Abstract
Flavonoid biosynthesis is characterized by extremely high flexibility; and it occurs in plants with different adaptive strategies in different habitats. The article proves that the flexibility of flavonoid biosynthesis has a fundamental nature and is the result of two circumstances: the special organization of the pathways of flavonoid metabolism and the relatively low specificity of the main enzymes of biosynthesis.
Keywords
Flavonoids, antioxidant activity, biosynthesis of flavonoids, Western Siberia, fractal analysis, enzymes
Introduction
Researchers are well aware that the accumulation of flavonoids in plants is characterized by high flexibility and has a poorly predictable nature. One of the reasons for it is that the flavonoid biosynthesis pathways are a complex network; in some areas allowing plants to biosynthesize the same flavonoid in several alternative ways (Harborne 1980; Kimura 1983; Rosenberg 1984; 2013; Tilman 2004; Gaston 2005; Hubbell 2006; Lambers et al. 2008; Mc Gill 2010; Petrussa et al. 2013; Mierziak et al. 2014). Fig. 1 shows the metabolic pathways of the main flavonoids and the stages of site formation that provide these molecules with antioxidant activity.
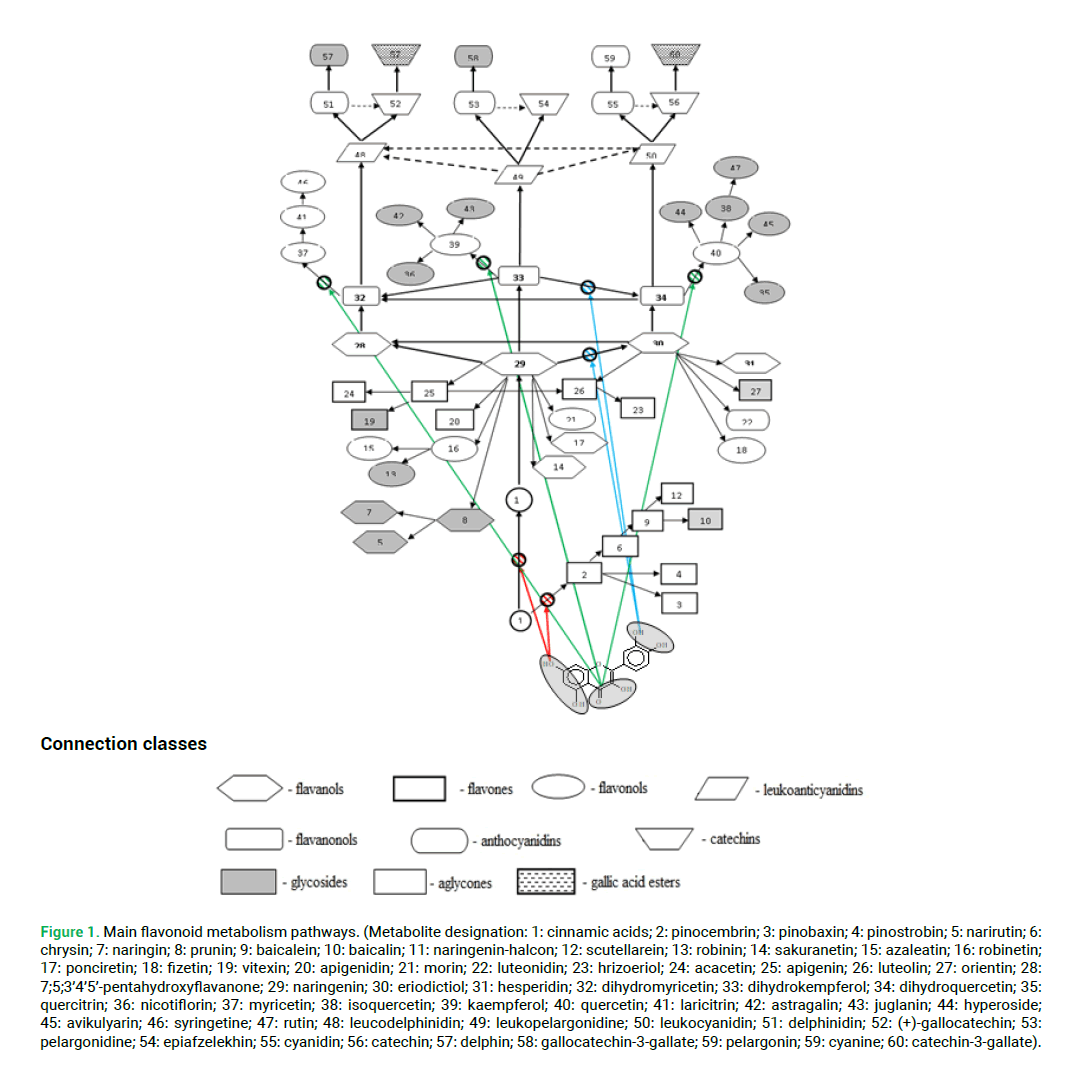
Figure 1: Main flavonoid metabolism pathways. (Metabolite designation: 1: cinnamic acids; 2: pinocembrin; 3: pinobaxin; 4: pinostrobin; 5: narirutin; 6: chrysin; 7: naringin; 8: prunin; 9: baicalein; 10: baicalin; 11: naringenin-halcon; 12: scutellarein; 13: robinin; 14: sakuranetin; 15: azaleatin; 16: robinetin; 17: ponciretin; 18: fizetin; 19: vitexin; 20: apigenidin; 21: morin; 22: luteonidin; 23: hrizoeriol; 24: acacetin; 25: apigenin; 26: luteolin; 27: orientin; 28: 7;5;3’4’5’-pentahydroxyflavanone; 29: naringenin; 30: eriodictiol; 31: hesperidin; 32: dihydromyricetin; 33: dihydrokempferol; 34: dihydroquercetin; 35: quercitrin; 36: nicotiflorin; 37: myricetin; 38: isoquercetin; 39: kaempferol; 40: quercetin; 41: laricitrin; 42: astragalin; 43: juglanin; 44: hyperoside; 45: avikulyarin; 46: syringetine; 47: rutin; 48: leucodelphinidin; 49: leukopelargonidine; 50: leukocyanidin; 51: delphinidin; 52: (+)-gallocatechin; 53: pelargonidine; 54: epiafzelekhin; 55: cyanidin; 56: catechin; 57: delphin; 58: gallocatechin-3-gallate; 59: pelargonin; 59: cyanine; 60: catechin-3-gallate).
The possibility of such alternative biosynthesis of the same molecules in plants is directly determined by the specifics of the involved enzymes. The study of the specifics of the main enzymes of flavonoid biosynthesis is the particular work objective of this paper.
Materials and Methods
The objects were samples of individual chromatograms of extracts of above-ground parts of 8 plant species of the Urals and Western Siberia. Samples of the above-ground parts of the plants from which the extracts were obtained were collected in various cenopopulations, differing in habitat conditions (Tab. 1).
Species name | Community | Habitat |
---|---|---|
Atriplex patula | Festuco-Puccinellietea | Stepes and salt flats of the Bashkir Trans-Urals |
Bassia seidoides | Festuco-Puccinellietea | Stepes and salt flats of the Bashkir Trans-Urals |
Kochia prostrata | Festuco-Puccinellietea | Stepes and salt flats of the Bashkir Trans-Urals |
Yuniperus sabina | Festuco-Brometea; Polygono-Artemisietea Austriacae |
Steppes and hills Bashkir Trans-Urals |
Glycyrrhiza korshinskii | Festuco-Puccinellietea | Stepes and salt flats of the Bashkir Trans-Urals |
Oxycoccus palustris | Oxycocco-Sphagnetea | Oligotrophic Swamps of Western Siberia |
Chamaedaphne calyculata | Oxycocco-Sphagnetea | Oligotrophic Swamps of Western Siberia |
Andromeda polifolia | Oxycocco-Sphagnetea | Oligotrophic Swamps of Western Siberia |
Table 1. Community classes and habitat locations of the studied species.
To measure the flavonoids, alcohol extracts were chromatographed on a Luna C18 250 mm × 4.6 mm, 5 μm column in a reverse-phase system. Flavonoids were measured in the above-ground organs of licorice and juniper by HPLC. The analysis used the Sigma-Aldrich standards: baicalein, hesperetin, fisetin, naringin, naringenin, rutin, quercetin, isoquercetin, morin, dihydroquercetin, and liquiritigenin 92% minimum purity. Flavonoid standards and the substances in samples were detected at 275 nm and 360 nm on a diode-matrix UV analyzer. Standards and substances in specimens were detected at 275 nm.
Results
The direct results of measuring the content of flavonoids in the samples were presented by the authors in previously published articles (Usmanov et al. 2019, 2020). In our studies, it was proved that the accumulation of flavonoids in the abovedescribed species is characterized by high flexibility. However, it was also found that the process of changing priorities in favor of the biosynthesis of particular flavonoids is in principle not strictly determined by either external or internal conditions. Fractal analysis revealed that the content of flavonoids in plants as a whole is a polyvariant multi-level system, in most cases having the properties of self-similarity (Ivanov et al. 2019; Usmanov et al. 2015, 2019, 2020).
In our opinion, such a polyvariant principle of flavonoid accumulation is impossible without a special scheme for organizing the work of the involved enzymes.
The main metabolic pathways described in the literature and the enzymes involved in the biosynthesis of the compounds under investigation (the corresponding ciphers are indicated in parentheses) and intermediate metabolites are presented below (BioCyc Pathway 2021):
Baicalein: 4-coumaric acid (EC 5.5.1.6)-pinocembrin (EC 1.14.20.5)-chrysin (EC 1.14.13)-baicalein.
Hesperetin: 4-coumaric acid (EC 6.2.1.12)-4-coumaroyl-CoA (EC 2.3.1.74)- naringenine-chalcone (EC 5.5.1.6)-naringenin (EC 1.14.14.82)-eriodictiol (EC 2.4.1.185)-hesperetin 7-O-B-D-glucoside (EC 2.4.1.236)-hesperetin.
Dihydroquercetin (Taxifolin): 4-Coumaric Acid (EC 6.2.1.12)-4-Coumaroyl-CoA (EC 2.3.1.74)-Naringenine Chalcone (EC 5.5.1.6)-Naringenin (EC 1.14.14.82)-Eriodictyol (EC 1.14 .11.9)-dihydroquercetin.
Quercetin: 4-coumaric acid (EC 6.2.1.12)-4-coumaroyl-CoA (EC 2.3.1.74)- naringenine-chalcone (EC 5.5.1.6)-naringenin (EC 1.14.14.82)-eriodictiol (EC 1.14.11.9)-dihydroquercetin (EC 1.14.20.6)-quercetin.
Morin: 4-coumaric acid (EC 6.2.1.12)-4-coumaroyl-CoA (EC 2.3.1.74)- naringenine-chalcone (EC 5.5.1.6)-naringenin (EC 1.14.19.76)-morin.
Naringenin: 4-coumaric acid (EC 6.2.1.12)-4-coumaroyl-CoA (EC 2.3.1.74)- naringenine-chalcone (EC 5.5.1.6) - naringenin.
Naringin: 4-coumaric acid (EC 6.2.1.12)-4-coumaroyl-CoA (EC 2.3.1.74)- naringenine-chalcone (EC 5.5.1.6)-naringenin (EC 2.4.1.185)-prunin (EC 2.4.1.236)- naringin.
Rutin: 4-coumaric acid (EC 6.2.1.12)-4-coumaroyl-CoA (EC 2.3.1.74)- naringenine-chalcone (EC 5.5.1.6)-naringenin (EC 1.14.14.82)-eriodictiol (EC 1.14.11.9)-dihydroquercetin 2.4.1.159)-rutin.
Fisetin: 4-coumaric acid (EC 6.2.1.12)-4-coumaroyl-CoA (EC 2.3.1.74)- naringenine-chalcone-(EC 5.5.1.6)-naringenin (EC 1.14.14.82)-eriodictyol (EC 1.14.19.76 )-fizetin.
Isoquercetin: 4-coumaric acid (EC 6.2.1.12.)-4-coumaroyl- CoA (EC 2.3.1.74)- naringenine-chalcone (EC 5.5.1.6)-naringenin (EC 1.14.14.82)-eriodictyol (EC 1.14.11.9 )-dihydroquercetin (EC 1.14.20.6)-quercetin (EC 2.4.1.91)-isoquercetin.
From the presented data, it follows that the enzymatic system of flavonoid biosynthesis in plants has some features:
• First, the same enzymes can simultaneously affect the biosynthesis of different flavonoids, especially when it refers to the synthesis of common precursors with their help. Thus, the 4-coumarate-CoA ligase enzyme (EC 6.2.1.12) is the basic since it begins the B-ring biosynthesis.
• Second, due to the relatively low specificity, some enzymes can perform the same operations with different classes of flavonoids (Tab. 2).
Table 2. The main enzymes involved in flavonoid biosynthesis.
EC Number | Enzyme | Reaction to carry out | Flavonoids, in the synthesis of which the enzyme is involved |
---|---|---|---|
1.14.11.9 | Flavanone-3-β-hydroxylase | Conversion of flavanones to flavanonols | Derivatives of myricetin, kaempferol and quercetin |
1.14.13 | Oxygenase | The transformation of chrysin in baikalein by the addition of a hydroxyl group with the participation of NADPH | Baikalein, Baikalin, Scutellarein |
1.14.14.82 | Flavonoid 3'monooxygenase | Conversion of apigenin to luteolin by attaching a hydroxyl group at the 3 'position | Luteolin, chrysoeriol |
1.14.19.76 | Flavone synthase II | Oxygen-assisted conversion of flavanones to flavones | Fisetin, apigenin, luteolin |
1.14.20.5 | Flavonoid 3'-hydroxylase (flavone synthase I) | Converting flavanones to flavones | Baicalein, Quercetin, Eriodictiol, Taxifolin |
1.14.20.6 | Flavonol synthase | Conversion of flavanonols to flavonols | Kemferol, quercetin, myricetin and their derivatives |
2.3.1.74 | Naringenin-chalcone synthase | Conversion of 4-coumaric acid to naringenin-chalcone by adding acetyl Co-A and creating ring B | Naringenin-chalcone and all its derivatives |
2.4.1.91 | Flavonoid-3-O-Glucosyl-transferase | Attachment of glucose to flavonoids | Kempferol, quercetin, myricetin |
2.4.1.185 | Flavanone-7-O-glucosyl transferase | Glycosylation with glucose | Prunin, hesperetin glucosid |
2.4.1.236 | 1,2 rhamnosyltransferase | Rhamnose glycosylation | Naringin, hesperidin |
5.5.1.6 | Chalcone isomerase | Converting Chalcones to Flavanones | Liquiritigenin, pinocembrin, naringenin |
6.2.1.12 | 4-coumarate-CoA ligasа | Attachment of CoA to 4-coumaric acid and initiation of ring B synthesis | All flavonoids |
As a result, the general scheme of the organization of flavonoid biosynthesis is so flexible that plants have the possibility of very ease and multi-level regulation of the composition of flavonoids. At the same time, there are numerous possibilities for the biosynthesis of particular compounds and precursors of entire classes by various alternative pathways (Tab. 1 and Fig. 1). Thus, the principle of multiple adaptive reactions is implemented in relation to flavonoid metabolism (Usmanov et al. 2016, 2017, 2018, 2021; Mavletova-Chistyakova et al. 2017; Ivanov et al. 2016).
Phenolic metabolite: 1: cinnamic acid, 2: naringeninechalcone, 3: apigenin, 4: luteolin, 5: naringenine, 6: 7,5,3’4’5’-pentahydroxyflavanone (dihydrotricetin), 7: eriodictiol, 8: dihydromyricetin, 9: dihydrokempferol, 10: dihydroquercetin, 11: myricetin, 12: kaempferol, 13: quercetin, 14: leukodelfinidine, 15: leukopelargonidine, 16: leukocyanidin, 17: delphinidin, 18: (+)-gallokatekhin, 19: pelargonidin, 20: afzelechin, 21: cyanidin, 22: katekhin.
Enzyme: Chalcone synthase (CHS, ЕС 2.3.1.74); Calcone isomerase (CHI, EC 5.5.1.6); Eriodictyol, Naringenine, NADPH-O-oxidoreductase, taxifolin hydroxylase (EC 1.14.13); apigenin, eriodictyol, NADPH-O-oxidoreductase, Flavanoid-3’- Hydroxylase (F3’H) (EC 1.14.13.21); flavonsynthase I and II (FNS I and FNS II, EC 1.14.11.22); Flavonoid 3’-5’-Hydroxylase (F3’5’H) (EC 1.14.13.88); Chalcone Isomerase I (EC 5.5.1.6); Flavanone- 3-β-hydroxylase (EC 1.14.11.9); Flavonol synthase (FLS, EC 1.14.11.23); Dihydroflavonol 4-reductase (DFR, EC 1.1.1.219); Leukoanthocyanidine dioxygenase/Anthocyanidin synthase (LDOX/ANS, EC 1.14.11); Leucoanthocyanidin Reductase (LAR, EC 1.3.1.77); Anthocyanidin Reductase (ANR), hypothetical enzymes.
During the long-term studies of the composition of flavonoids of the above-mentioned species, it was found that the accumulation of these compounds, as well as their total content, are extremely variable (Usmanov et al. 2015, 2019, 2020; Ivanov et al. 2019). At the same time, no stable correlative relationships between the accumulation of particular compounds and the numerical values of environmental indicators were observed in all the species under investigation. It was found that the general picture of flavonoid accumulation in plants demonstrates the properties of an object of a multifractal nature (Gelashvili et al. 2013; Usmanov et al. 2016; Shcherbakov et al. 2021).
It is known that the anti-oxidative function is the most important and universal function of flavonoids in plants. Since the composition of flavonoids in plants is extremely flexible, the total potential antioxidant activity of accumulated flavonoids in particular plants can be achieved through a variety of options for the accumulation of these compounds (from a relatively small number of highly effective, to significant values of relatively low-effective substances) with all potential options for transition states. Thus, plants show the ability to achieve the same goal in a variety of alternative ways.
Such a polyvariance would again be impossible without special adjustment of the enzyme systems. As follows from Fig. 2, among the enzymes involved in the flavonoid biosynthesis, there are relatively universal substances that may perform the same transformations with substances of different classes. These are mainly enzymes belonging to class 1.14. (monooxygenases, oxidoreductases). This very enzymatic versatility allows plants to solve the problems of biosynthesis of particular flavonoids and their classes in the most diverse way after the biosynthesis of the common precursor naringenin (Fig. 2). For example, the number of the pathways of luteolin and myricetin biosynthesis can reach five options (Fig. 2).
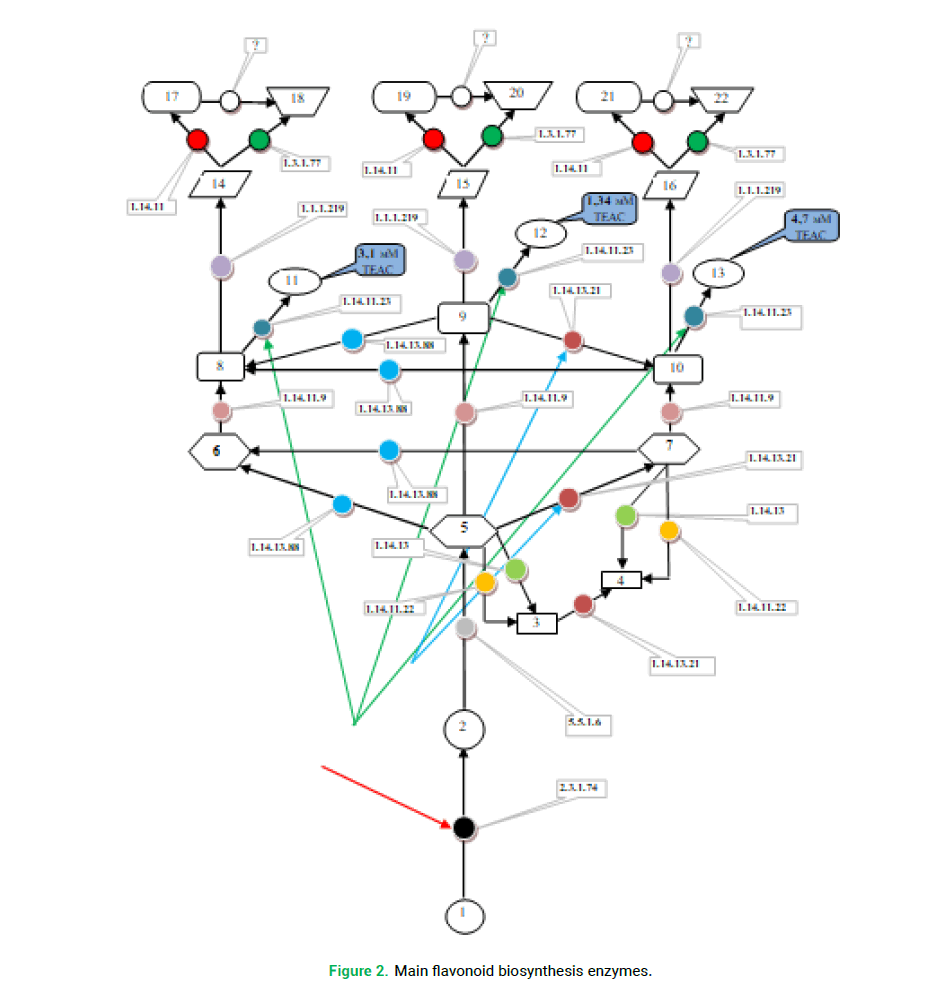
Figure 2: Main flavonoid biosynthesis enzymes.
Thus, the polyvariant nature of flavonoid biosynthesis in plants in response to the poorly predicted nature of the habitat is the result of the fact that a significant number of enzymes with relatively low specificity are involved in the metabolism of these substances.
Conclusion
The studies conducted over many years give us reason to believe that the flexibility of flavonoid accumulation is a fundamental property of plant metabolism and provided by the following circumstances:
1. The relatively low specificity of some of the most important enzymes allows these enzymes to perform the same transformations with different substances.
2. The system of flavonoid metabolism pathways is organized in such a way that allows the possibility of biosynthesis of the same substances by several alternative pathways.
3. Additional degrees of freedom are provided by a flexible system of modification of base molecules through the creation and decomposition of a large number of derivatives of base molecules, primarily glycosides.
Acknowledgments
The work was supported by the grant from the Russian Foundation for Basic Research (RFBR) 18-44-860006.
References
Harborne, J.B. (2013). The flavonoids: advances in research since 1980. https://slovar.cc/enc/bse/2022978.html
Kimura M. (1979). The neutral theory of molecular evolution. Scientific Amer 241: 98-129. https://www.jstor.org/stable/24965339
Rosenberg G.S. (1984). Modeli v fitocenologii. Мoscow. USSR: Publishing House Nauka 240.
Tilman D. (2004). Niche tradeoffs, neutrality, and community structure: a stochastic theory of resource competition, invasion, and community assembly. Proceedings of the National Academy of Sciences 101: 10854-10861.
Gaston K.J., Chown S.L. (2005). Neutrality and the niche. Functional Ecol 19: 1-6.
Hubbell S.P. (2006). Neutral theory and the evolution of ecological equivalence. Ecology 87: 1387-1398. https://doi.org/10.1890/0012-9658(2006)87[1387:NTATEO]2.0.CO;2
Lambers H., Chapin III F.S., Pons T.L. (2008). Plant physiological ecology. Springer Sci Business Media 605.
McGill B.J. (2010). Towards a unification of unified theories of biodiversity. Ecol Letters 13: 627-642. https://doi.org/10.1111/j.1461-0248.2010.01449.x
Petrussa E., Braidot E., Zancani M., Peresson C., Bartolini A., Patui S., Vianello A. (2013). Plant flavonoids-biosynthesis, transport and involvement in stress responses. Int J Mol Sci 14: 14950–14973. https://doi.org/10.3390/ijms140714950
Rosenberg G.S. (2013). Introduction to theoretical ecology. In two volumes; 2nd ed., revised and expanded. Togliatti: Cassandra 1: 565, 2: 445.
Mierziak J., Kostyn K., Kulma A. (2014). Flavonoids as important molecules of plant interactions with the environment. Molecules 19: 16240-16265. doi: https://doi.org/10.3390/molecules191016240
Usmanov I.Yu., Yumagulova E.R., Aleksandrova V.V., Ivanov S.P., Shcherbakov A.V., Ivanov V.B., Gonchar I.G. (2019). Fractal analysis of flavonoids in complex chemical compositions in extracts of Chamaedaphne calyculata (L.) Moench (ERICACEAE) in oligotrophic swamps of Western Siberia. Modern Phytomorphol 13: 35-40. http://doi.org/10.5281/zenodo.3518841
Usmanov I.Yu., Ivanov V.B., Abdrakhimova G.S., Shcherbakov A.V., Yumagulova E.R., Aleksandrova V.V. (2019). Fractal analysis of flavonoids in composition hplc-fingerprint extracts of Oxycoccus palustris Pers. (ERICACEAE) in oligotrophic swamps of Western Siberia. Internat J Adv Biotechnol Res 10: 369-376.
Usmanov I.Yu., Yumagulova E.R., Aleksandrova V.V., Gonchar I.G., Shcherbakov A.V., Ivanov V.B. (2019). Kompleksy flavonoidov Chamaedaphne Calyculata (l.) Moench oligotrofnyh bolot Srednej Obi. Vestnik Nizhnevartovskogo Gosudarstvennogo Universiteta 2: 59-71.
Usmanov Iskander, Ivanov Vyacheslav, Shcherbakov Arkadiy (2020). System for ecological regulation of the biosynthesis of flavonoids as a strange attractor. Modern Phytomorphol 14: 77-84. http://doi.org/10.5281/zenodo.4449908
Ivanov V.B., Shcherbakov A.V., Gonhar I.G., Ivanova A.V., Usmanov I.Yu. (2019). Using the principles of fractal analysis for description of plant flavonids metabolism. Internat J Adv Biotechnol Res 10: 456-464.
Usmanov I.Yu., Ovechkina E.S., Yumagulova E.R., Ivanov V.B., Shcherbakov A.V., Shayahmetova R.I. (2015). Problemy samovosstanovleniya ekosistem Srednego Priob'ya pri antropogennyh vozdejstviyah neftedobyvayushchego kompleksa. Vestnik Nizhnevartovskogo Gosudarstvennogo Universiteta 1: 79-86.
Usmanov I.Yu., Shcherbakov A.V., Ivanov V.B., Yumagulova E.R. (2019). Fraktal'nyj analiz sistemy biosinteza flavonoidov. V knige: IX S"ezd obshchestva fiziologov rastenij Rossii Fiziologiya rastenij-osnova sozdaniya rastenij budushchego. (Kazan', 18–24 sentyabrya 2019 g.): tezisy dokladov.-Kazan': Izdatel'stvo Kazanskogo universiteta 446.
Usmanov I.Y., Shcherbakov A.V., Ivanov V., Ivanov S., Gonchar I. (2020). Use of fractal analysis principles when describing flavonoids variety of the south trans-urals plants. Modern Phytomorphol 14: 13-19. https://doi.org/10.1016/j.still.2016.04.005
BioCyc Pathway. Genome Database Collection. https://biocyc.org/
Usmanov I.Yu., Shcherbakov A.V., Mavletova M.V., Yumagulova E.R., Ivanov V.B., Aleksandrova V.V. (2016). Pul'siruyushchaya mnogomernaya ekologicheskaya nisha rastenij: rasshirenie ob"ema ponyatiya. Izvestiya Samarskogo nauchnogo centra Rossijskoj akademii nauk. 18: 525-529.
Usmanov I.Yu., Yumagulova E.R., Ivanov V.B., Korkina E.A., Shcherbakov A.V., Ivanov N.A., Ryabuha A.V. (2016). Adaptaciya ekosistem Srednego Priob'ya v zone neftedobychi: ierarhiya i dlitel'nost' processov. Vestnik Nizhnevartovskogo Gosudarstvennogo Universiteta 2: 87-94.
Usmanov I.Yu., Yumagulova E.R., Ivanov V.B., Aleksandrova V.V., Ivanov N.A., Schaichmetova R.I., Scherbakov A.V., Mavletova-Chistuakova M.V. (2017). Physiological barriers for adventitious species invasion in oligotroph ecosystems of the middle Ob Area. Vegetos: Interntion J Plant Res 30: 81-85. http://dx.doi.org/10.5958/2229-4473.2017.00195.1
Usmanov I.Yu., Ivanov V.B., Ivanov N.A. (2018). Samovosstanovlenie ekosistem Srednego Priob'ya pri antropogennyh vozdejstviyah neftedobyvayushchego kompleksa. V sb.: Ekologicheskie problemy bassejnov krupnyh rek-6: Materialy mezhdunarodnoj konferencii. Tol'yatti: Anna. 303-305.
Mavletova-Chistyakova M.V., Shcherbakov A.V., Ivanov V.B., Yumagulova E.R., Usmanov I.Yu. (2017). Pul'siruyushchaya mozaichnost' parametrov pochv Yuzhnogo Zaural'ya. Vestnik Nizhnevartovskogo Gosudarstvennogo Universiteta 4: 124-133.
Ivanov V.B., Alexandrova V.V., Usmanov I.Y., Yumagulova E.R., Chibrikov O.V., Ivanov N.A., Shcherbakov A.V. (2016). Comparative evaluation of migrating anthropogenic impurities in ecosystems of the middle Ob region through bioindication and chemical analysis. Vegetos 29: 43-46. http://dx.doi.org/10.5958/2229-4473.2016.00018.5
Usmanov I.Yu., Scherbakov A.V., Ivanov V.B., Ivanova A.V. (2021). Fractal nature of multidimensional ecological niche: real habitats in south trans-urals. In S.G. Maximova, R.I. Raikin, M.M. Silantyeva, A.A. Chibilyov (Eds.). Advances in Natural, Human-Made, and Coupled Human-Natural Systems. Springer.
Gelashvili D.B., Iudin D.I., Rozenberg G.S., Yakimov V.N., Solntsev L.A. (2013). Fractals and multifractals in bioecology. Nizhniy Novgorod 370.
Usmanov I.Yu., Yumagulova E.R., Ovechkina E.S., Ivanov V.B., Aleksandrova V.V., Ivanov N.A., Shcherbakov A.B. (2016). Fractal analysis of morpho-physiological parameters of oxycoccus polustris pers in oligotrophic swamps of Western Siberia. Vegetos: Internat J Plant Res. 29: 1-3. https://doi.org/10.5958/2229-4473.2016.00002.1
Scherbakov A.V., Ivanov V.B., Ivanova A.V., Usmanov I.Y. (2021). The equifinal achievement of the total antioxidant activity of flavonoids by plants in various habitats. IOP Confer Series: Earth Environ Sci 670: 012018.