Research Article - Modern Phytomorphology ( 2025) Volume 19, Issue 1
Development of whitefly-resistant cotton by transforming the Tma12 gene isolated from Tectaria macrodonta
Farah Naz1*, Arfan Ali2, Ghulam Zahara Jahangir3, Usman Arif1, Qurban Ali4, Muhammad Tariq1, Abdul Munim Farooq1 and Idrees Ahmad Nasir12FB Genetics, Four Brothers Group, Lahore, Pakistan
3Centre for Applied Molecular Biology (CAMB), University of The Punjab, Lahore, Pakistan
4Department of Plant Breeding and Genetics, Faculty of Agricultural Sciences, University of The Punjab, P.O BOX. 54590, Lahore, Pakistan
Farah Naz, Centre of Excellence inMolecular Biology, University of the Punjab, Lahore, Pakistan, Email: farah.naz@cemb.edu.pk
Received: 12-Feb-2025, Manuscript No. MP-25-161231; , Pre QC No. MP-25-161231 (PQ); Editor assigned: 14-Feb-2025, Pre QC No. MP-25-161231 (PQ); Reviewed: 28-Feb-2025, QC No. MP-25-161231; Revised: 14-Apr-2025, Manuscript No. MP-25-161231 (R); Published: 21-Apr-2025
Abstract
Cotton is white gold and contributes significantly to the economy of the countries. The process of evolution of resistance in various cotton insects directly affects the life of people. Among the cotton insect pests, the sucking pests have turned out to be destructive. The sap sucking insects especially whitefly (Bemisia tabaci) has emerged as major agricultural pests. So, the efforts are now being engaged in improving Genetically Modified (GM) cotton varieties resistant to whitefly. None of the insecticidal proteins used in Genetically Modified (GM) crop plants to date are effective against whitefly. Some insecticidal proteins showed significant toxicity against the whiteflies such as Hvt, lectin, and Tma12 proteins. The identified Tma12 protein from an edible fern, Tectaria macrodonta that is insecticidal to whitefly and interferes with its life cycle. But still the understanding of the biological function of fern genes and their potential uses for agriculture, environment and health has been hampered by the lack of high-quality genome sequence of fern. In this study, gene cassette comprising CAMV35S promoter, Tma12 gene isolated from Tectaria macrodonta and NOS terminator respectively was synthesized and cloned in the plant expression vector. This respective gene cassette was transformed via agrobacterium-mediated transformation. The putative transgenic plants were screened through conventional PCR. Moreover, the mRNA expression of the Tma12 gene was in five plants which was 3-fold higher in transgenic cotton line as compared to the non-transgenic cotton plants. Finally, the insect bioassay with five transgenic cotton lines showed up to 70% mortality against whitefly. Study is important as it will enhance resistance of local triple gene cotton variety against whitefly. This novel insecticidal protein fulfils the need to combat resistance build-up against sucking pests especially whitefly.
Keywords
Genetically modified crops, Tma12 gene, Tectaria macrodonta, Whitefly
Introduction
Naturally cotton plant is shrubby and cultivated generally in subtropical and tropical regions (Viot et al., 2023). It is shrubby plant which grows all around warm regions of the world like America, India and Africa. G. hirsutum alone is responsible for 80% of the total Asian production. This crop contributes 60% to the total fibre of the world and has become very important crop (Tariq et al., 2023). Pakistan is the fifth largest producer of cotton. Pakistan is in top three countries which export raw cotton and at fourth position in consumption of it but this position is under threat now due to diseases, viruses and weather changes (Sain et al., 2023). In national context, cotton brings foreign exchange in the form of exported yarn and textile products of considerable amount for Pakistan. But cotton is under strong biotic stress in the country. During 2015-16 season alone, the cotton growers sustained huge loss of 27.65%. A total of 10.1 million bales were produced in that year as compared to 2014-15 in which Pakistan produced 13.96 million bales (Iqbal et al., 2023). For many decades cotton plants are normally targeted by numerous production challenges from which majority are of biotic including pests and viruses. Cotton the most precious agricultural crop is being damaged worldwide by more than 10,000 species of insects/pests (Mohamed et al., 2023). These pests, insects represent considerable direct or indirect yield loss. The commercialization of synthetic pesticides, in the middle of last century, fundamentally transformed traditional strategies of crop protection. Their remarkable immediate efficacy, their ease of use and their relatively low cost when compared with the benefits obtained, ensured their rapid and widespread adoption. This yield protection, for the first time, allowed growers to exploit fully on modern techniques of varietal selection, fertilization and irrigation and to come close in capturing the full genetic yield potential of the selected varieties (Gojon et al., 2023). The mechanisms by which ferns and mosses resist insects are not well understood. Ferns produce phytoecdysones that severely impair insect development and cause molting abnormalities. Cotton today takes over 18% of world insecticide use and significant proportions of other pesticides globally. To circumvent the yield losses due to insect attack and environmental hazard due to pesticides (Ambreen et al., 2023), diverse approaches are evolving for genetic engineering of crops to impart host plant derived pest resistance.
Genetic engineering in the field of molecular biology gained a significant progress in the last three decades to overcome the hurdles posed by conventional breeding techniques (Ai et al., 2023). After that GM crops including glyphosate resistant soybeans, Bt cotton, Bt maiz, and Bt potato were allowed to grow. A total of 529 transgenic crops were reported at that time. Asian countries such as China, India, Pakistan, and Bangladesh grow significant GM crops in which Pakistan was the grower of maize and cotton. (ISAAA Database, 2021). Recently Brazil approved the wheat event HB4 for commercial cultivation on 3rd March 2023. Sucking insects (whitefly, thrip, aphid, jassid, and mealybug) damage the cotton crop in two ways. Firstly, these insects suck the essential plant nutrients especially from leaf and develop a fungus named sooty mold on the surface of the leaf that results in wilting, yellowing, drying of the plant and reduced fibre quality (Chandrasekhar et al., 2017). Secondly, these insects also act as carriers of hazardous plant viruses such as Begomoviruses transmitted by whitefly in a circulate manner (Czosnek et al., 2017). Cotton crop plants are no exception when it comes to susceptibility to these sucking, chewing and virus delivering insects.
There is an amazing fact that still none of the effective transgenic strategy is available to combat yield loss by infestation of sap-sucking pests including mired bugs, aphids, whitefly, thrips and leafhoppers (Kiruthika et al., 2022). Population dynamics of sucking pests in dual season cotton ecosystem and its correlation with weather factors. White fly infest several hundred crop species including economically important crops, so called polyphagous insects (Soumia et al., 2021). Especially whitefly damages the crop by sucking phloem sap. The damage is in the form of premature defoliation, stunted growth, early wilting etc. Another indirect plant damage by whitefly is excreted honey dew which facilitates fungal growth on fruit surfaces and on leaves resulting in the reduced product market value. More than 200 viruses infesting crops are being transmitted by whitefly resulting complete yield loss (Navas-Afzal et al., 2023). None of the insecticidal proteins used in Genetically Modified (GM) crop plants to date are effective against whitefly. Significant reduced pesticide usage in Bt cotton crop increases the frequency of unaffected pests including whitefly. And so, damage have been increasing continuously. (Malinga et al., 2024). Whitefly acts as vector and helps in the transmission of the viral diseases. CLCV occur due to consistent sucking of whitefly (Sarwar et al., 2023). None of the insecticidal proteins used in Genetically Modified (GM) crop plants to date are effective against whitefly. The identified Tma12protein from an edible fern, Tectaria macrodonta that is insecticidal to whitefly (median lethal concentration 1.49 mg/ml in in vitro feeding assays) and interferes with its life cycle at sublethal doses (2023). Transgenic crops resistant to whiteflies are desirable because the technology could be supplied as seeds (Giri et al., 2013). Tma12 gene was selected to control whitefly. Tma12 is a protein from an edible fern, Tectaria macrodonta that is insecticidal to whitefly. None of the insecticidal proteins used in Genetically Modified (GM) crop plants to date are effective against whitefly. Tma12 is a protein which Interferes with whitefly life cycle. But still the understanding of the biological function of fern genes and their potential uses for agriculture, environment and health has been hampered by the lack of high-quality genome sequence of fern. These novel insecticidal proteins fulfills the need to combat resistance build-up against sucking pests. Development of transgenic crops expressing Bt toxins and Tma12 insecticidal fern protein has provided effective plant protection against these insect pests.
Materials and Methods
Tma12 gene characterization
Nucleotide sequences from Gene Bank for Tma12 GenBank: JQ438776.1. An online tool, Expert Protein Analysis System (ExPASY) was used for the analysis of open reading frames (ORFs) of these selected gene sequences (Shukla et al, 2016). Also these genes sequences were codon optimized specifically for Gossypium hirsutum cotton spp. for high transgene expression using freely web-based available tool (integarated DNA Technologies web port) (owczaryz et al., 2008). Gene under specific CAMV 35S promotor were synthesized chemically by bio-basic Inc. Tma12 gene cassette of 1582 bp with fast digest restriction endonucleases SmaI and kpnI cutting sites.
Restriction analysis through digestion of pCAMBIA 2300
The plant binary vector pCAMBIA2300 vector was digested with the Fast Digest SmaI and Fast Digest KpnI. The reaction mixture for this step had a volume of 0.02 mL and has acquainted as outlined in Tab. 1. The concluded incubation was at 37°C for 300 seconds.
Components for Tma12 gene | Volume |
---|---|
Plasmid DNA (500 ng) | 4 µl |
Sma1 | 1 µl |
Kpn1 | 1 µl |
FD Green Buffer | 2 µl |
H2O | 12 µl |
Total | 20 µl |
Table 1. Reaction mixture preparation for restriction digestion of pCAMBIA2300.
Map of Tma12 gene cassette in pCAMBIA2300 vector is as shown below
Expression construct for transgenic activity of Tma12 gene having cAMV35S promoter 678bp, 651 bp Tma12 gene and 253 bp NOS terminator and with Fast Digest SmaI and Fast Digest kpnI restriction digestion sites in pCAMBIA2300 vector was designed as followings (Fig. 1).
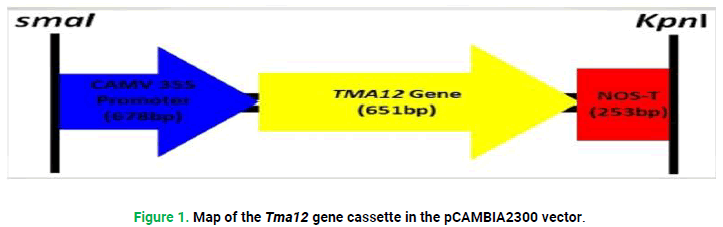
Figure 1: Map of the Tma12 gene cassette in the pCAMBIA2300 vector.
Cloning of the Tma12 cassette into the pCAMBIA2300 vector
The overhangs of the gene cassette (insert) and pCAMBIA2300 was ligated together with T4 enzyme DNA Ligase of Thermo Scientific Rappid DNA Ligation Kit, Cat#K1242 in accordence to the manufacturer’s instructions. Both vector and insert were measured for concentration with Qubit Nanodrop ND-1950. The vector to insert ratio was adjusted to be 1:1 and 1:3 respectively. Insert concentration was 90 ng/ul The Ligation mixture was incubated at 22° C for one hour.
Transformation of Gossypium hirsutum
De-linted seeds must be used for transformation of specific genes. So, for this purpose, soaked the seeds of the local cotton cultivar in concentrated H2SO4 100 ml/kg with unremitting stirring of 4-5 minutes although observing that the whole lint is detached, and the shiny surface of the seeds appeared. After this, all seeds must be completely splashed with tap water. 5-6 washings were more than enough with continuous removal of floater seeds. Required seeds must be air dried completely before starting further procedure. Cotton seeds must be surface sterile in laminar flow hood before the process of germination began. Surfactants of 5% HgCl2 and 10% SDS with autoclaved water were used for seeds washing. Come to assure that the complete removal of two detergents and floater seeds was accomplished by continuous 10-15 washings of plenty of autoclaved water. Only sinker seeds were selected for germinate in glass flasks with adequate amount of autoclaved water on 30°C almost for two days.
A. Tumefaciens inoculum preparation
Streaking of positive agrobacterium cells were made on agar YEP plates with kanamycin of 50 μg/mL and rifampicin of 100 μg/mL concentration containing for Tma12 gene. Incubated these plates at 30°C for two days. An inoculum of well separated single colony was prepared with selected drugs using 10 ml YEP broth and incubated in a rotary shaker for two days at 30°C. After getting growth the bacterial cells were pellet down at 4000 rpm centrifugation for 15 minutes and dissolved in 10 mL of MS broth.
Isolation of embryos
Well germinated seeds in glass flasks were taking to laminar hood. Testa on seeds and cotyledonary leaves were excised by using sterile forceps and surgical blade. Put the seeds for recombinant gene transformation on the laminar flow hood. Isolated mature embryos were placed on wet filter paper in laminar air flow to avoid contaminations before the initiation of transformation.
Agrobacterium mediated transformation (shoot apex method)
The genetic transformation of cotton seeds was done by the shoot-apex-cut method as described by (Ali et al., 2016). After selecting germinated embryos, these embryos were made cut at their plumule site with the help of sterile sharp blade and then added to the A. tumefaciens culture in MS broth medium. Further, incubated this media for 2 hours with continuous shaking at 30°C. After taking out embryos from broth media, they were desiccated by placing them on autoclaved filter paper. Further, cut embryos were then placed on plates containing MS medium.
Acclimatization of the plant
Transferred emerging embryos from MS media containing petri plates to test tubes when true roots of these plants appeared. Then enclosed these test tubes with cotton plugs. When the transgenic cotton plants were 4-6 weeks, were considered completely routed. Plants with better growth results were selected. Wash away the roots with non-ionic water which also contained fungicide, dried with tissue paper. So carefully transferred these rooted plantlets to untainted soil containing pots. This pure soil fertilizer contains equal proportion of clay, sand and peat moss (1:1:1 while minute quantity of fungicide was also mixed to check fungal attack. (Sriram et al., 2021). To maintain humidity, the plants in pots must be covered with polythene bags. Maintained the room temperature for these pots in growth room at 28 ± 2 °C. Also maintained a photoperiod of 16 hours’ light and 8 hours’ dark under light intensity of 250 μmol m-2 S-1-300 μmol m-2 S-1. However, water and fresh air were occasionally provided to them by removing the polythene bags briefly. After 4-5 days of shifting to soil, plants gradually got adjusted to changed environment. In the beginning, plants were placed to sun light for duration of 10-15 minutes and increased that time duration up to 15 minutes on daily basis. When plants started to pick this measured change, this period was extended to reach 6 hours daily. After maintaining performance in the presence of sun light, they were planted in field.
Molecular analysis at T° generation
Genomic DNA extraction: Leaves of Tma12 transgenic cotton plants were then subjected to isolate genomic DNA for molecular study. Two different protocols were subjected to analyse the transgenic cotton plants (Shukla et al., 2016). PCR was done using the following set of gene specific primers with the product size 588bp (Tab. 2).
Gene specific primer | Primer Sequence | Product size |
---|---|---|
Tma12 CEMB, Forward primer | 5`GCAACAGCGTTACATGGGGT3` | 588 |
Tma12 CEMB, Reverse primer | 5`CAATGGGCTACTGCTAACTT3` |
Table 2. Gene specific primers for Tma12.
Gene expression examination through real-time PCR
RNA extraction from transgenic cotton plants: Total RNA from transgenic cotton leaves was extracted using a modified protocol as described by (Jaakola et al., 2001). Total RNA was isolated by taking 1 g of young leaves of transgenic cotton leaves. Liquid nitrogen in pestle mortar was used to ground the samples. Shifted the homogenized tissues to labeled falcon tubes. Pre heated RNA extraction buffer (1 ml) was added into it. Incubated this homogenate for 15-20 minutes at 65°C and vortexed for at least 3 times during incubation after every 5 minutes. Added chloroform and isoamyl alcohol (24:1) and mixed thoroughly. Centrifuged the samples at 4000 rpm for 20 min. carefully separated out the supernatant in fresh labeled 1.5 mL tubes without disturbing central protein layer. Added 10M LiCl of 60% concentration. Incubated the samples at 4°C overnight to precipitate RNA completely. Following centrifuged the tubes at 13000 rpm (4°C) for 30 minutes. Discarded the supernatant and washed pellet twice with 70% ethanol. Air-dried the pellet and dissolved in 100 μL of preheated SSTE buffer. The pellet was again mixed in an equal volume (100 μL) of phenol: chloroform: isoamylalcohol (25: 24: 1) and then with an equal volume of chloroform: isoamylalcohol (24: 1) for more purification. In the supernatant added two volumes absolute ice-cold ethanol. Incubated the samples at -20°C for overnight. After that centrifuged the samples at 13000 rpm for 20 minutes at 4°C. Pellet was collected and washed with 70% ethanol, air-dried and resuspended in DEPC treated water.
Real-time PCR: By using primer3 online software gene specific primers were synthesized for expression analysis of Tma12 genes. Primer sequences used for RT-PCR are given below (Tab. 3).
Primer name | Primer sequence | Expected product size |
---|---|---|
Tma12-F | CTGCTGTTTTGCATCCTGAA | 300 bp |
Tma12-R | AATCGCACTTTCCACCGTAA | |
GAPDH-F | CACGGCCACTGGAAGCACAC | 101 bp |
GAPDH-R | TCCTCAGGGTTCGTGATGCC |
Table 3. Primer sequences for real time PCR with their product sizes.
The quantitative real-time qRT-PCR reaction was carried out in an iQ5 cycler (BIORAD) with a 96-well plate. To perform RT-PCR, protocol of Maxima SYBR Green/ROX (Cat# K0229) was followed. For every DNA specimen triplicate reaction for RT-PCR was prepared. To prepare reaction mixture of total 20 μl volume following components were added; the plate of 96 wells was covered with septa and to mix all the components very well centrifugation was performed. All this process was carried out on ice. For internal control reference gene, the housekeeping gene, Glyceraldehyde3-Phosphatedehydrogenase (GAPDH), was used to normalize the data.
Insect bioassay on whitefly
Insect bioassay with Bamisia tabaci was performed under controlled conditions as T1 transgenics (6 weeks old stage) including fresh Tma12 transgenic and non-transgenic cotton plants using methodology reported earlier (Jin et al., 2011) were grown in a glass house at 36 ± 1° C, 14L/10D and ≈ 61% humidity. The plants were left un-infested for one week by carefully isolating them in a net cage. The whitefly culture was maintained on non-transgenic cotton plants in a separate greenhouse at same conditions. Before the start of bioassay, the 0-24 hr old whitefly adults were captured from the maintained culture and kept on ice to minimize environmental stress. The ice-inactivated whiteflies were carefully released into Tma12 transgenic plant lines (in triplicates) leaves also taken in replicates. The net cage /mesh bags facing the three biological replicates from each transgenic and control cotton lines were used in the bioassay. The mortality data was recorded on third day of infestation. One-way Analysis of Variance (ANOVA) was performed to compare the significance mortality levels between transgenic and non-transgenic control lines (Dunnett’s test) at 95%. (P<0.005) confidence level using GraphPad prism software version 5 for windows (Prism 2007).
Fluorescence In situ Hybridization (FISH)
The confirmation of transgene integration in the cotton genome was done by Fluorescence In situ Hybridization (FISH) technique to locate Tma12 gene on the chromosomes. For this purpose, probe was labeled with the label IT nucleic acid labeling kit (Mirus Bio LLC), Cy3, following instructions as described in manual. In situ hybridization was carried out by following protocols as described by Noreen et al. On metaphase chromosomal spreads. Fluorescent signal was detected by using a fluorescent microscope (Olympus Model BX6l) and fluorescent signals were detected by the using of blue (DAPI) and red. For capturing fluorescent signals and analyzed by using Genus 3.7 software by Cytovision applied imaging system. The same software package was utilized for karyotyping.
Statistical analysis
One-way Analysis of Variance or ANOVA (Dunnett’s test) and two-way ANOVA (Tukey’s test) were run to analyse the significance level between non-transgenic control and transgenic cotton lines at 95% confidence interval. Software used for above analysis was Graph Pad Prism (version 5).
Results
Cloning of Tma12 gene cassette in pCAMBIA2300
Tma12 gene cassette assembly was cloned in pCAMBIA2300 binary vector through propagating in E.coli Top 10F’ cells. Then picked randomly six transformed colonies and cultured them. Good quality mini-prep for plasmid yield from six cultures were subjected on examination for the presence of Tma12 gene cassette assembly. Colony PCR with gene specific primers of six isolated plasmids resulted the presence of ~588 bp construct in expression vector (Fig. 2b). Tma12 gene cassette assembly's exact fragment size was determined by restriction digestion using the Fast Digest SmaI and Fast Digest kpnI restriction enzymes (Fig. 2a). Providing evidence confirmed the Tma12 gene cassette assembly's inclusion in the pCAMBIA2300 vector's adoption for agrobacterium transformation.
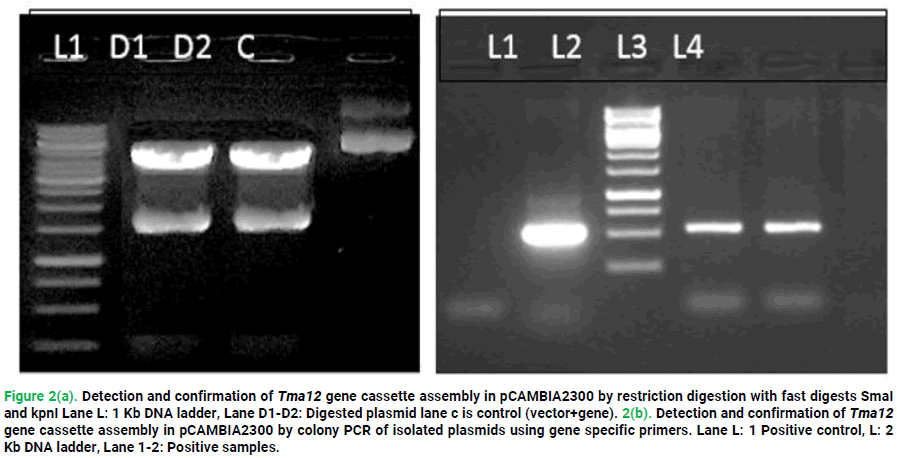
Figure 2. 2(a). Detection and confirmation of Tma12 gene cassette assembly in pCAMBIA2300 by restriction digestion with fast digests SmaI and kpnI Lane L: 1 Kb DNA ladder, Lane D1-D2: Digested plasmid lane c is control (vector+gene). 2(b). Detection and confirmation of Tma12 gene cassette assembly in pCAMBIA2300 by colony PCR of isolated plasmids using gene specific primers. Lane L: 1 Positive control, L: 2 Kb DNA ladder, Lane 1-2: Positive samples.
Electroporation of pCAMBIA-Tma12 gene cassette into A. tumefaciens
Tma12 gene cassette was successfully electroporated to transfer recombinant pCAMBIA-tma12 construct to A. tumefaciens (strain LBA4604). Gene specific primers in a PCR further confirmed the effective analysis of construct transfer to produce transgenic progeny.
Agarose electrophoresis explains the 588 kb fragment confirmed positive colonies as shown in Fig. 2b.
Agrobacterium-mediated transformation using the shoot apex technique
For transformation of local variety of Gossypium hirsutum, a locally developed and commercially approved cotton variety, planned 22 cotton seed transformation experiment sets which were completed within two months of the time. Explants of developed embryos were reserved to progress this experiment. Roughly, 2748 healthy embryos were utilized in transformation by pCAMBIA-Tma12 recombinant-agrobacterium cloned through tissue culturing via co-cultivation and to develop transgenic cotton as already mentioned in material & methods chapter. Cut the epicotyl ends of healthy embryos and exposed those with inoculum of agrobacterium comprising pCAMBIA_Tma12 construct. To develop the shots and roots, plantlets were transferred to MS media containing glass tubes for additional 48 days. When roots appeared in MS media it’s the time to shift the plant in soil pots (Fig. 4). So,sixty three plants were competent and shifted to soil pots. The general transformation proficiency remained 0.7%. The steps wise process of transformation shown in Fig. 3.
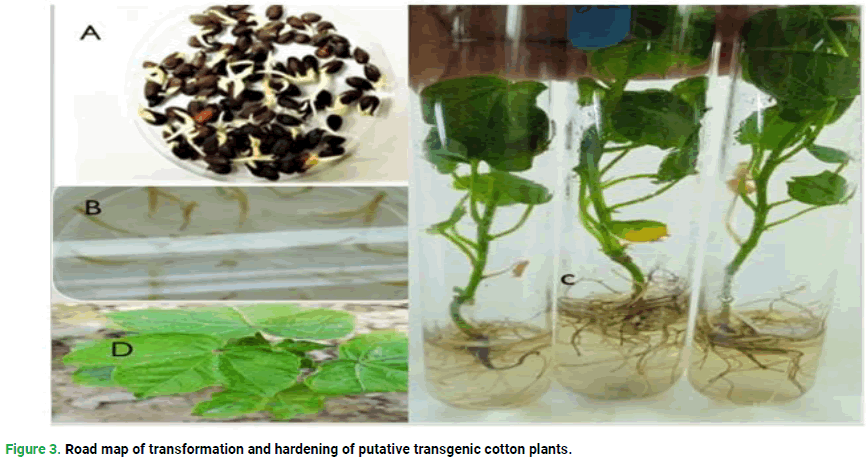
Figure 3. Road map of transformation and hardening of putative transgenic cotton plants.
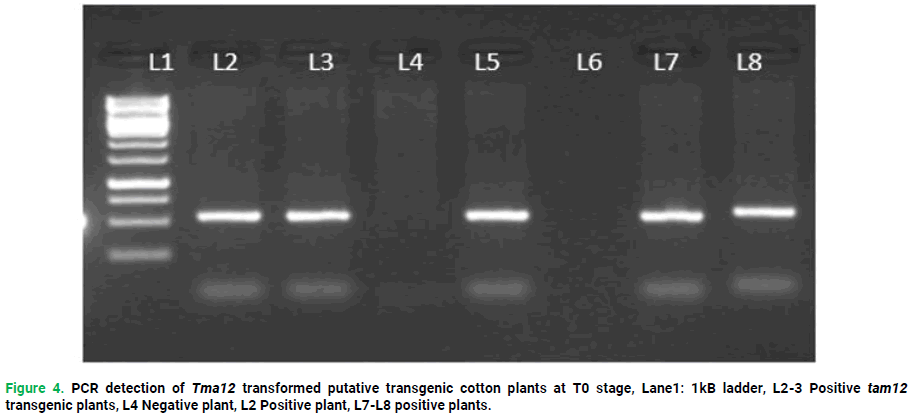
Figure 4. PCR detection of Tma12 transformed putative transgenic cotton plants at T0 stage, Lane1: 1kB ladder, L2-3 Positive Tma12 transgenic plants, L4 Negative plant, L2 Positive plant, L7-L8 positive plants.
Transformation efficiency for T° transgenic cotton plants
Out of 2748 early grown embryos only 200 were successfully germinated and transferred to MS media. After acclimatization and further screening over PCR, only17 Tma12 transgenic cotton plants (L1P3, L1P6, L1P9, L1P10, L2P12, L2P15, L2P17, L2P20, L3P22, L3P26, L4P27, L4P28, L8P47, L9P52, L10P54, L10P55, L15P57. in comparison to typical plants, these mentioned plants had a strong positive resilience and normal developmental profile. Transgenic T0 cotton plants were used to describe the transgenic lines of Tma12. Transformation efficiency, 0.7% was achieved overall.
Real-Time quantitative PCR (RT-qPCR)
Real-Time quantitative PCR (RT-qPCR) was performed to quantify the transcript level of tma12 in primary transformants. Total RNA was extracted by CTAB method as illustrated by Zhao. cDNA was synthesized from 1μg RNA by using RevertAid First Strand cDNA Synthesis Kit (Thermo Scientific) and followed Kit’s manual. For qPCR, gene specific primers and for internal control GAPDH primers were used. The reaction mixture contained 1X Maxima SYBR Green, 1 μM of each primer, RNAse-free sterile water and cDNA template. The rest of the procedure was followed as earlier described by Riaz et al., (2023) and qPCR reaction conditions were one cycle at 95°C for 5min, 35 cycles at 95°C for 30s, 60°C for 30s and 72°C for 30s. The Cq values of all the samples were analyzed through PikoRe al™ Software 2.1 (Thermo Scientific) (Fig. 5).
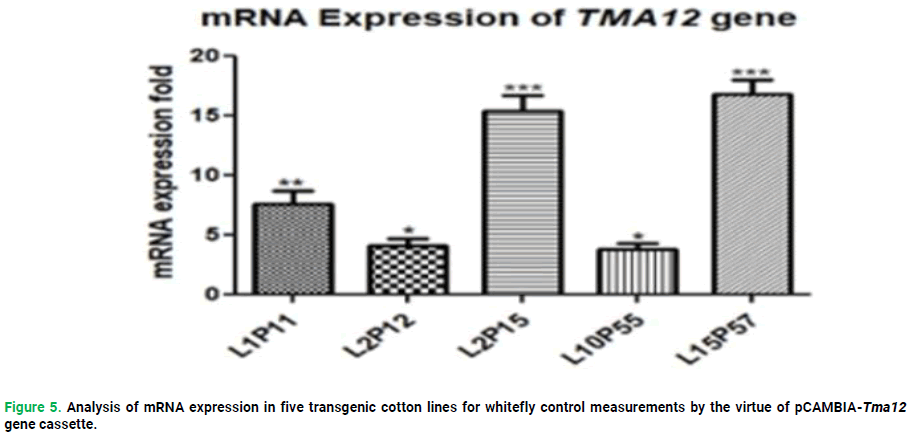
Figure 5. Analysis of mRNA expression in five transgenic cotton lines for whitefly control measurements by the virtue of pCAMBIA-Tma12 gene cassette.
Evaluation of bioengineered crops resistance against the insects
Five Tma12 transgenic cotton lines were selected for their toxicity evaluation against whitefly (Bamisia tabaci) 1-3 instar and adult whitefly in T1 progeny by a modified pots bioassay. For bioassay of whitefly selected five best transgenic cotton lines and a non-transgenic plant in triplicates covered with mesh bags. On each individual transgenic cotton line and control, a total count of 30 whiteflies (1-3 instar and adults) were introduced. Dislodged the mesh bags from each plant after 24 hours of feasting on Tma12 transgenic the insects initiated a notable changed behavior like odd stretching of the corpus. On non-transgenic cotton lines, the whitefly inoculum was thriving and productive. Mortality information was compiled for 72 hours or three days complying with the occurrence of the infestation. Heavy colonization of Whitefly was detected on non-transgenic plant under leaves (Fig. 6A). Almost 372 per control plant of immature and adult whiteflies was on an average as shown in the Fig. 6B. T1 transgenic lines were highly non-susceptible against different steps of whiteflies lifecycle (only 31/plant) observed in Fig.6B & C. Transgenic cotton lines L1P1,1 L15P57 and L2P15, showed 85%; 79%, 69% mortality respectively. Using the non-transgenic cotton line as the control, transgenic lines were tested by ANOVA with 99% confidence intervals at P 0.001 and P 0.0001 respectively (Tab. 4).
Total average count of immature and adult whiteflies on five T1 transgenic plants selected as 3 replicates for each transgenic plant, and 3 control as non-transgenic negatives. It appears from the figure that transgenic plant L15P57, which has highest expression of Tma12 gene (by the virtue of pCAMBIA_Tma12 transformation), and no virus symptoms showed (least average number of whiteflies (immature+adult) i.e., 31, followed by plant L2P15 with 43 whiteflies alive and L1P11 with 50 alive whiteflies in comparison with the control negative line of the same cotton cultivar which had 280 counts of whitefly at p<0.5. Control plant susceptible to whitefly showing symptoms of whitefly sucking and CLCV (carried by whitefly). In-set of both pictures, one can see the back sides of the leaves from both plants clearly signifying the effect of pCAMBIA_Tma12 transformation.
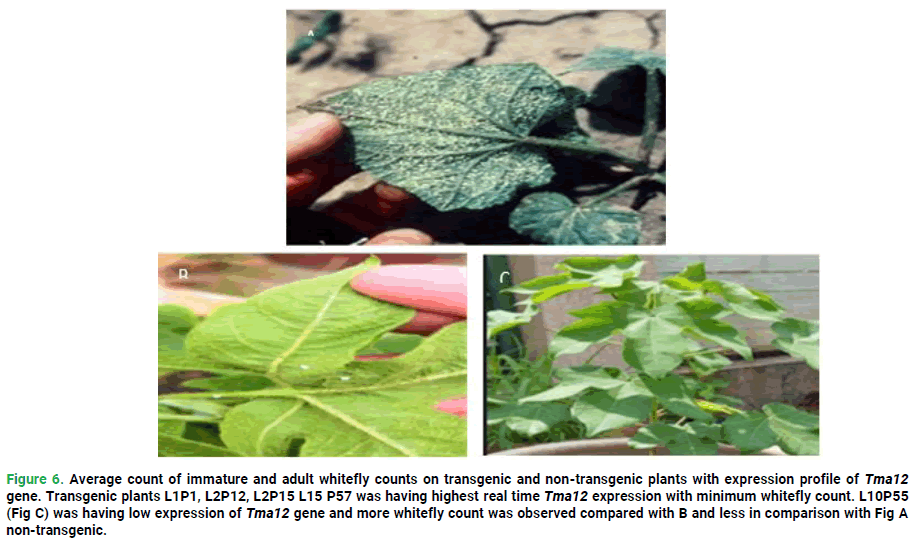
Figure 6. Average count of immature and adult whitefly counts on transgenic and non-transgenic plants with expression profile of Tma12 gene. Transgenic plants L1P1, L2P12, L2P15 L15 P57 was having highest real time Tma12 expression with minimum whitefly count. L10P55 (Fig C) was having low expression of Tma12 gene and more whitefly count was observed compared with B and less in comparison with Fig A non-transgenic.
Plant | Whitefly population immature | Adult | Average whitefly population (Immature+Adult) | Reduction |
---|---|---|---|---|
L1P11 | 45 | 39 | 107 | 9 fold |
L1P11 | 91 | 62 | ||
L1P11 | 84 | 54 | ||
L2P12 | 49 | 30 | 98 | 6.1 fold |
L2P12 | 29 | 29 | ||
L2P12 | 44 | 34 | ||
L2P15 | 50 | 22 | 77 | 4.59 fold |
L2P15 | 39 | 35 | ||
L2P15 | 55 | 21 | ||
L10P55 | 29 | 30 | 45 | 3.28 fold |
L10P55 | 22 | 45 | ||
L10P55 | 39 | 55 | ||
L15P57 | 88 | 37 | 117 | 12 fold |
L15P57 | 156 | 67 | ||
L15P57 | 176 | 85 | ||
Untransformed A | 266 | 140 | 363 | - |
Untransformed B | 223 | 108 | ||
Untransformed C | 213 | 125 |
Table 4. Average count of white fly infestation.
Location and transgene copy number determination
One Tma12 T1 transgenic cotton line L15P57 was subjected to karyotyping analysis which locates transgene and copy number at different phases of developing cells; prophase; interphase and metaphase through specific probe mechanism. The Tma12 gene T1 transgenic cotton plant transgenic line L15P57 exhibited homozygosity at metaphase, showed one copy at 13th chromosome for Tma12 gene (Fig. 7B). In the non-transgenic control cotton plant, there was no signal (Fig. 7A).
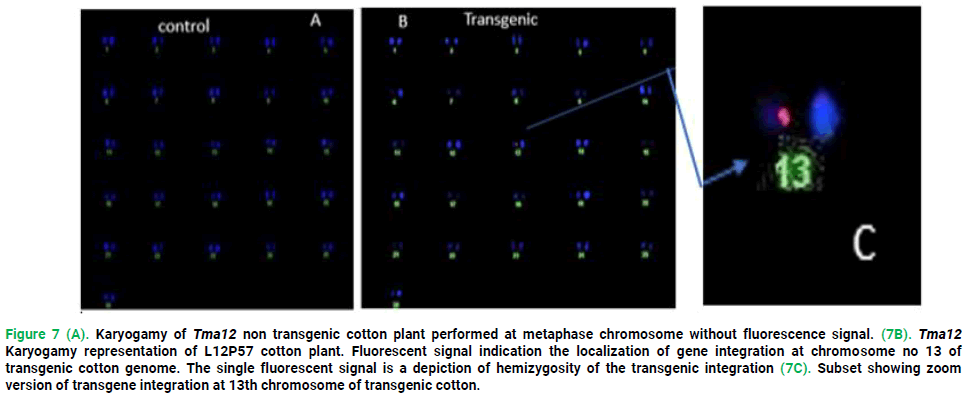
Figure 7. Karyogamy of Tma12 non transgenic cotton plant performed at metaphase chromosome without fluorescence signal. (7B). Tma12 Karyogamy representation of L12P57 cotton plant. Fluorescent signal indication the localization of gene integration at chromosome no 13 of transgenic cotton genome. The single fluorescent signal is a depiction of hemizygosity of the transgenic integration (7C). Subset showing zoom version of transgene integration at 13th chromosome of transgenic cotton.
Discussion
The most important cash crop is the cotton by which the farmers living is being enhanced and cotton is also responsible for 80% industrial raw material to and 30% of foreign exchange generaters. By 2027, the global market for cotton is predicted to reach US$ 46.56 billion. India, China, Pakistan, and the United States are the four largest cotton producers in the world. (Ashraf et al., 2018). The global cotton trade will be expected to increase by around 7% between 2022-2023 compared to previous year. It is predicted to maintain its rising trend, increasing by 1.6% annually or nearly in line with growth in mill use (http://www.depts.ttu.edu/aaec/icac/). Sucking and chewing insects provide the greatest hazard to cotton in Pakistan. These two insect groups are thought to be responsible for between 50 and 60 percent of total yield losses (Arora et al., 2018). Whitefly is very devasting pest lowers the quantity and the quality as well of cotton. Whitefly infestation causes about 50% lowering of yield (Zhang et al., 2020). In year 2019, Pakistani cotton growing sector sustained a loss of 6 million bales worth approx. 3 billion USD denting already struggling national economic recession (Chohan et al., 2023). Experts blame abiotic and biotic factors, including irregular weather patterns and sucking/chewing/virus pests respectively, for these agronomically calamitous conclusions. Cotton is under strong biotic stress; whitefly is the crucial one hitting the cotton with its extensive sap-sucking and mediating septicity of Cotton Leaf Curl Virus (CLCuV) (Mafakheri, 2020). Plant viruses, like other viruses, are obligatory intracellular parasites that depend only on the resources of their host cells to replicate. Serious plant illnesses are caused by plant viruses that infect plants. In the current study, an effort was made to develop transgenic cotton plants through the introduction of Tma12 gene to minimize losses caused by the infestation of major sucking insect whitefly.
In the very first of my study, we will discuss the insecticidal aspect of Tma12 gene against whitefly. The Tma12 insecticidal gene was transformed into a local cotton cultivar with pCAMBIA2300 vector under CaMV35S promoter. The purpose of the study was to evaluate the expression of the respective gene in cotton and its toxicity to sucking insect (immature-adult whitefly) bioassay. A natural necessity arose during last five years to develop a cotton plant that would be resistant or at least tolerant to some satisfactory degree besides whitefly infestation. Conventional breeding techniques require extensive time period to develop desired trait in plants which is over-run resultantly annoy the degree of demand. Therefore, non-transgenic cotton plants were planned to genetically modified by executing the cotton plants infection with a bacteria called A. tumefaciens in this research. There could be number of options in the process of selecting candidate genes from Cry or Lectins or other pest-toxic gene families but, considering its efficiency, ability to defy multiple biotic stresses and short sequence, Tma12 gene from edible fern, Tectaria macrodonta was chosen and transfigured. The cloning and orientation of synthetic Tma12 gene cassette in binary plant expression vector pCAMBIA2300 was verified through polymerase chain reaction and restriction digestion. The recombinant vector (pCAMBIA2300-Tma12) was then successfully electroporated in Agrobacterium tumefaciens and confirmed through PCR and restriction analysis. Pitzschke and Hirt (2010) used similar cloning procedure to localize the expression of Cry1Ac and Cry2Ac proteins in the chloroplast agrobacterium, in several monocotyledonous and many dicotyledonous plants. The recombinant vector was transformed into a locally developed cotton variety through shoot apex cut method of Agrobacterium mediated transformation. Shoot apical meristems isolated from 4500 mature cotton seedlings as explants were co-cultivated with Agrobacterium tumefaciens strain LBA 6404 harboring synthetic gene cassettes. A total of sixty-three putative transgenic cotton plants were obtained and shifted to the field after initial screening. The overall transformation efficiency remained at 1.07%. The similar approach was also used by (Rao et al., 2011b) in cotton and got transformation efficiencies in the range of 1%-1.0% in cotton. 1 also used similar approaches in cotton transformation experiments and got transformation efficiencies in the range of 1%-1.0%. Jin et al., (1990) explains the transfer of the T-DNA in another experiment. The successful transformation in putative transgenic cotton plants was confirmed through different molecular techniques. The amplification of 588 bp fragment of Tma12 gene in seventeen-putative transgenic cotton lines (L1P3, L1P6, L1P9, L1P10, L2P12, L2P15, L2P17, L2P20, L3P22, L3P26, L4P27, L4P28, L8P47, L9P52, L10P54, L10P55, L15P57) were observed. Out of total sixty-three plants that were shifted to field, using gene specific internal primers, confirmed the successful introduction of Tma12 gene in cotton. The results were in correlation with (Vergunst et al., 2000). The mRNA expression of Tma12 fern origin insecticidal gene was taken through quantitative real-time qRT-PCR in five T1 transgenic cotton lines (L1P11, L2P12, L2P15, L10P55, L15P57). The comparative analysis of Ct values obtained by qRT-PCR analysis revealed that the mRNA expression of Tma12 gene varied from 8-12 folds in the lines L15P57 and L10P55 respectively as depicted in. The results were in consistent with (Wou et al., 2011) in which the authors produced transgenic cotton plants with synthetic Tma12 gene fused with chloroplast 91 transit peptide and achieved three-fold increase in expression level of Tma12 and hence proved to be highly toxic against wide range of sucking insects.
Fluorescent In situ Hybridization (FISH) was preferred than Southern hybridization in determining the transgene location and copy number in the cotton genome due to its reliability and accuracy studied that cytogene analysis using Fluorescence In situ Hybridization (FISH) and resulted that FISH is a powerful tool for the analysis and physical mapping of the genome. Ling et al., (2010) resulted the important global view of the gene insertion and location of transgene on the chromosome depicting the structure of individual and whole genomes in vivo, given that the cytogenetic approach is based on the analysis of intact chromosomes. The transgene expression is directly related to different factors like promoter, insertion point of genes, copy number and location on host also depicted the same research work as well. One plant from five highest mRNA expressed T1transgenic cotton lines was subjected for FISH at different stages of cell division using Tma12 specific probe following the same procedure as explained by (Rao et al., 2013). The L15P57 transgenic cotton plant showed homozygosity (one copy numbers) on chromosome number 13th at late metaphase stage whereas, no signal was observed in the non-transgenic control cotton plant. The results are in accordance with (Ali et al., 2016) who evaluated two cotton varieties (CRSP-I and CRSP-II) for the expression and location of Cry1Ac+Cry2A, GTG-gene and compared the genes expression level and resistance against insects relative to copy number of genes. In conclusion, there has been significant protection level achieved against whitefly (Bemisia tabaci) in cotton plants as a result of nuclear transformation and expression of the Tma12 gene. The resultant transformed cotton lines may be enrolled in breeding programs with high-yielding cotton varieties for further improvement in production characteristics. Similar findings reported by Raybould and Vlachos (2011), also confirmed our results that expression of the Tma12 gene does not have any unintended impact on overall morphology and phenotypic characteristics of the transgenic plant.
The final objective of the current study was to evaluate transgenic cotton plants expressing the fern insecticidal Tma12 gene under a constitutive promoter (CaMV35S) against whitefly (Bamisia tabaci) in the advanced T1 generation. The confirmed transgenic cotton plants showing higher expression of Tma12 in T1 generation were selected for insect bioassay. Among other transgenic strategies using gene families including but not limited to cry toxinns, this is the unique study that reports whitefly tolerance in cotton plants during a very facilitative hot and humid season (for all sucking insects) by the virtue of Tma12 gene expression. Almost 78% to 85% mortality of whiteflies (Bamisia tabaci) as compared to non-transgenic control, confirmed significant resistance development in transgenic cotton plants against whitefly (Bamisia tabaci) expressing fern modified insecticidal protein (Fig. 4). Besides the minor damage to the leaves of transgenic cotton line L10P55, all other lines remained undamaged. All the pupa and mature whitefly that survived after the 72 hrs of infestation on transgenic line L15P57 were extremely weak and a significant delay in the growth of whitefly nymph was observed as compared to the whitefly nymph fed on non-transgenic cotton plants, the results were in complete accordance with (Kumar et al., 2023). The transgenic cotton lines L15P57, L2P15, L2P12 and L1P11 showed 89%; 86%, 85%, and 80% mortality rates of whitefly in 72 hrs insect-bioassay in controlled environment grown transgenic cotton plants as compared to non-transgenic control plants where the mortality was 10% which can be due to some natural stress (Fig. 4). Similarly, all the dead insects showed notable physical changes like the abnormal stretching of the body likely due to the expression of fern insecticidal protein that targets the life cycle of whitefly (Javaid et al., 2016).
Conclusion
The current research work was proposed to utilize and evaluate the insecticidal spectrum of fern origin Tma12 protein in the cotton plant to enrich the insecticidal potential of the transgenic cotton plants against major insects specifically sucking insects. Insect bioassays using viruliferous whiteflies adults and nymphs used confirmed the efficacy of transgenes. Significant mortality of sucking insects on transgenic cotton plants tells the success story of this study. The study is a part of future strategy to overcome resistance build-up in insects due to extensive use of Bt toxins against specific receptors. By using next generation Bt toxins having different target receptors will be helpful in delaying resistance and making longer and stable insect control which will impact on national economy and farmers.
References
Afzal M, Saeed S, Riaz H, Ishtiaq M, Rahman MH. (2023). A critical review of whitefly (Bemisia tabaci Gennadius) cryptic species associated with the cotton leaf curl disease. J Innov Sci. 9:24-43. [Crossref] [Google Scholar]
Ai TN, Bui APN. (2023). Synteny of cotton SSR markers genomes paves the way for resistance against black root rot disease in cotton. Adv Life Sci. 9:462-466. [Crossref] [Google Scholar]
Ali A, Ahmad S, Nasir IA, Rao AQ, Husnain T. (2016). Evaluation of two cotton varieties CRSP1 and CRSP2 for genetic transformation efficiency, expression of transgenes Cry1Ac+ Cry2A, GT gene and insect mortality. Biotechnol Rep. 9:66-73. [Google Scholar] [Crossref] [PubMed]
Ambreen F, Hashmi MAH, Abbas S. (2023). Genotoxic response of Oreochromis niloticus exposed to tertiary mixture of pesticides. Adv Life Sci. 9:384-390. [Crossref] [Google Scholar]
Arora R, Kataria SK, Singh P. (2017). Breeding for insect resistance in cotton: advances and future perspectives. In: Breed Insect Resist Crops Sustain Agric. 265-288. [Crossref] [Google Scholar]
Ashraf S, Sangi AH, Hassan ZY, Luqman M. (2018). Future of cotton sector in Pakistan: A 2025 outlook. Pak J Agric Res. 31:23. [Crossref] [Google Scholar]
Awan ZA, Sufyan F, Mehdi SA. (2023). Assessment of seed priming effect on germination and cotton productivity of two cotton varieties in Multan. Adv Life Sci. 9:552-559. [Crossref] [Google Scholar]
Chandrashekar K, Shashank PR. (2017). Indian contribution to whitefly (Bemisia tabaci) research. In: Century Plant Virol India. 563-850. [Crossref] [Google Scholar]
Chauhan JS, Waghmare VN, Singh KH. (2023). Cotton crop in India: growth, varietal improvement and seed production. 37:1-15. [Google Scholar]
Czosnek H, Hariton-Shalev A, Sobol I, Gorovits R, Ghanim M. (2017). The incredible journey of Begomoviruses in their whitefly vector. Viruses. 9:273. [Crossref] [Google Scholar]
Giri NH, Singh R, Taggar GK. (2023). Screening technique to evaluate soybean resistance to whitefly, Bemisia tabaci (Gennadius) under screen-house conditions. J Entomol Res. 47:309-316. [Crossref] [Google Scholar]
Gojon A. (2023). Approaches and determinants to sustainably improve crop production. Food Energy Secur. 12:369. [Crossref] [Google Scholar]
Iqbal J, Faheem M, Roman M, Magsi S, Rauf H, Arif Z. (2023). Climate change effects on cotton planting date and planting density using modelling techniques. Pure Appl Biol. 12:732-753. [Crossref] [Google Scholar]
Global status of commercialized biotech/GM crops: 2018. 2018. [Google Scholar]
Jaakola L, Pirttilä AM, Halonen M, Hohtola A. (2001). Isolation of high quality RNA from bilberry (Vaccinium myrtillus L.) fruit. Mol Biotechnol. 19:201-203. [Crossref] [Google Scholar] [PubMed]
Kiruthika A, Murugan M, Jeyarani S, Sathyamoorthy NK, Senguttuvan K. (2022). Population dynamics of sucking pests in dual season cotton ecosystem and its correlation with weather factors. Int J Environ Clim Change. 12:586-595. [Crossref] [Google Scholar]
Kumar A, Suroshe SS, Saini GK, Singh J. (2023). Efficacy of genetically transformed Metarhizium anisopliae against Spodoptera litura and Aphis craccivora. Saudi J Biol Sci. 30:103493. [Crossref] [Google Scholar] [PubMed]
Ling A, Cordaux R. (2010). Insertion sequence in versions mediated by ectopic recombination between terminal inverted repeats. PLoS One. 5:15654. [Crossref] [Google Scholar] [PubMed]
Mafakheri M, Kordrostami M. (2020). Role of molecular tools and biotechnology in climate-resilient agriculture. In: Plant Ecophysiol Adapt under Clim Change: Mech Perspect. II. 491-529. [Crossref] [Google Scholar]
Malinga LN, Laing MD. (2024). A review of pesticides for the control of some cotton pests. Ann Agric Crop Sci. 9:1148. [Crossref] [Google Scholar]
Mohamed NH, Ali AMH, Tawfik AI. (2023). Feeding deterrence and larvicidal effects of latex serum and latex-synthesized nanoparticles of Calotropis procera against the cotton leafworm, Spodoptera littoralis. Adv Life Sci. 10:585-592. [Crossref] [Google Scholar]
Rao AQ, Din S, Akhtar S. (2016). Genomics of salinity tolerance in plants. In: Plant Genom. Intech. 273-299. [Google Scholar]
Raybould A, Vlachos D. (2011). Non-target organism effects tests on Vip3A and their application to the ecological risk assessment for cultivation of MIR162 maize. Transgenic Res. 20:599-611. [Crossref] [Google Scholar] [PubMed]
Sain SK, Brown JK, Monga D. (2023). Global status of cotton leaf curl disease–An ongoing threat to cotton production. In: Cotton: Some Insights Crop Improv Soc India. 92-134. [Google Scholar]
Sarwar M, Saleem MF, Ali B. (2023). Manipulation of plant growth stimulants on plant morphology, phenology, and disease incidence of Gossypium hirsutum L. under various thermal regimes. Arab J Geosci. 16:170. [Crossref] [Google Scholar]
Shukla AK, Upadhyay SK, Mishra M. (2016). Expression of an insecticidal fern protein in cotton protects against whitefly. Nat Biotechnol. 34:1046-1051. [Crossref] [Google Scholar]
Soumia PS, Pandi GP, Krishna R, Ansari WA, Jaiswal DK, Verma JP, Singh M. (2021). Whitefly-transmitted plant viruses and their management. In: Emerging Trends in Plant Pathology. 175-195. [Crossref] [Google Scholar]
Sriram S, Ramanujam B. (2021). Formulations and delivery systems. In: Biopestic Hortic Crops. 41. [Google Scholar]
Tariq M, Khan MA, Muhammad W, Ahmad S (2023). Fiber crops in changing climate. In: Glob Agric Prod: Resil Clim Change Cham: Springer Int Publ. 267-282. [Crossref] [Google Scholar]
Viot CR, Wendel JF. (2023). Evolution of the cotton genus, Gossypium, and its domestication in the Americas. Crit Rev Plant Sci. 42:1-33. [Crossref] [Google Scholar]
Wu KM, Lu YH, Feng HQ. (2008). Suppression of cotton bollworm in multiple crops in China in areas with Bt toxin–containing cotton. Science. 321:1676-1678. [Crossref] [Google Scholar] [PubMed]
Zhang XM, Lövei GL, Ferrante M, Yang NW, Wan FH. (2020). The potential of trap and barrier cropping to decrease densities of the whitefly Bemisia tabaci MED on cotton in China. Pest Manag Sci. 76:366-374. [Crossref] [Google Scholar] [PubMed]